Project
our goals
Imaging and remote sensing protocols in the classical domain are fundamentally limited by the diffraction limit and detection noise. To move beyond these boundaries photonic quantum technologies provide new paradigms for achieving unprecedented sensing performance. The SURQUID project will achieve both super-resolution below the Rayleigh diffraction limit and super-sensitivity below the shot noise limit for light detection and ranging (lidar) applications.
Using quantum homodyne detection (QHD) combined with non-classical illumination using entangled coherent states (ECS) we will realize a quantum lidar system for multi-scale quantum imaging with unparalleled accuracy and precision. We will implement a long-term stable QHD system by integrating multiple superconducting single photon detectors (SNSPDs) in nanophotonic circuits.
Operation in the telecommunication spectral window, where atmospheric transparency is high, will enable remote quantum imaging on a logarithmic length scale from 100 mm to 100 km distances. Waveguide-integrated SNSPDs excel in performance in the telecom wavelength range and provide a scalable route towards multi-wavelength and multi-detector architectures. We will implement a two-color quantum lidar system where time-of-flight (TOF) detection with ultralow timing jitter below 10 ps on one wavelength will give information about target distance with 2 mm accuracy, while QHD combined with ECS illumination on a second wavelength will provide quantum-enhanced local spatial resolution. Through beam scanning and synchronized QHD we will realize super-resolved surface profiling.
Our consortium is uniquely placed to tackle these challenges by joining leading experts in ultrafast single photon detection, nanophotonic circuit design and quantum light generation. The SURQUID project will realize a ready-to-use quantum lidar system for applications in super-resolved object identification, remote sensing and quantum enhanced imaging.
our research
Source of entangled coherent states
Differing from today’s single photon sources, which have emerged primarily from quantum optics applications, our novel chip-based SURQUID ECS source will provide non-classical states which can be employed outside a laboratory setting. The integrated ECS source will provide key technological advances beyond the quantum sensing application, e.g. For continuous variable quantum computation, gravitational interferometry, and quantum enhanced precision measurements. We will realize an optical parametric amplifier (OPA) pumped from a frequency-doubled pulsed C-band laser. The corresponding wavelengths (~1550 nm) are an optimal compromise between ranging resolution, high atmospheric transmittance, and interfacing with nanophotonic circuits and waveguide-coupled superconducting nanowire single-photon detectors (SNSPDs). Depending on the channel loss the source will be tuned via the pump parameter settings and fast switching to yield coherent states (CS), N00N-states, non-Gaussian entangled (or MM’) states or entangled coherent states (ECS). Within the project all these states will be experimentally verified (e.g., via violation of Bell inequalities or similar entanglement criteria). The source will be implemented in a portable form factor (prototype) for deployment in the field.
Source of entangled coherent states
Differing from today’s single photon sources, which have emerged primarily from quantum optics applications, our novel chip-based SURQUID ECS source will provide non-classical states which can be employed outside a laboratory setting. The integrated ECS source will provide key technological advances beyond the quantum sensing application, e.g. For continuous variable quantum computation, gravitational interferometry, and quantum enhanced precision measurements. We will realize an optical parametric amplifier (OPA) pumped from a frequency-doubled pulsed C-band laser. The corresponding wavelengths (~1550 nm) are an optimal compromise between ranging resolution, high atmospheric transmittance,
and interfacing with nanophotonic circuits and waveguide-coupled superconducting nanowire single-photon detectors (SNSPDs). Depending on the channel loss the source will be tuned via the pump parameter settings and fast switching to yield coherent states (CS), N00N-states, non-Gaussian entangled (or MM’) states or entangled coherent states (ECS). Within the project all these states will be experimentally verified (e.g., via violation of Bell inequalities or similar entanglement criteria). The source will be implemented in a portable form factor (prototype) for deployment in the field.
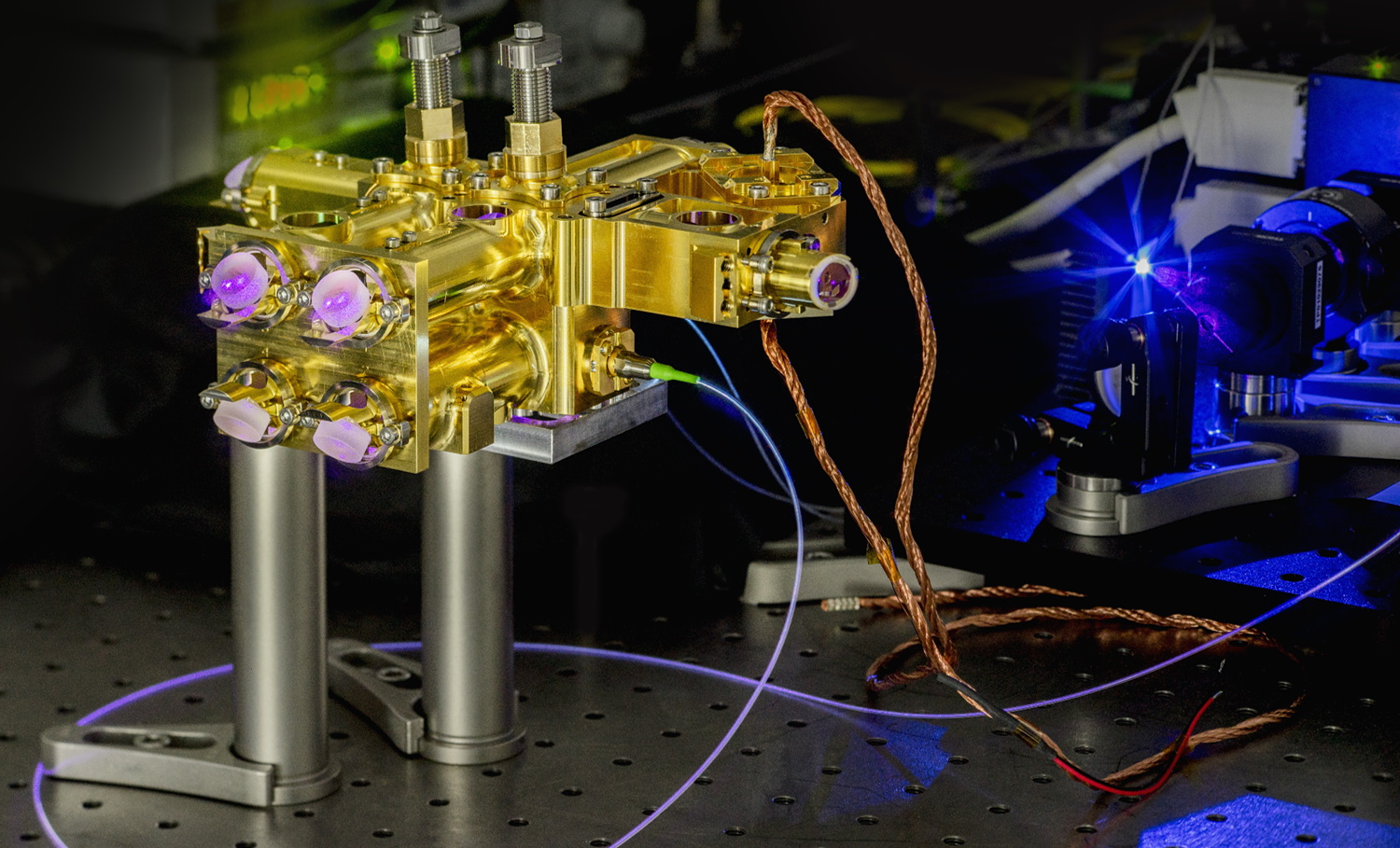
Space EPS by Fraunhofer IOF
Space suitable entangled photon source for satellite based QKD, emitting up to 300k pcs @ >98% fidelity and 5 mW of pump power. Project funding by the European Space Agency and the German government is appreciated.
Space EPS by Fraunhofer IOF
Space suitable entangled photon source for satellite based QKD, emitting up to 300k pcs @ >98% fidelity and 5 mW of pump power. Project funding by the European Space Agency and the German government is appreciated.
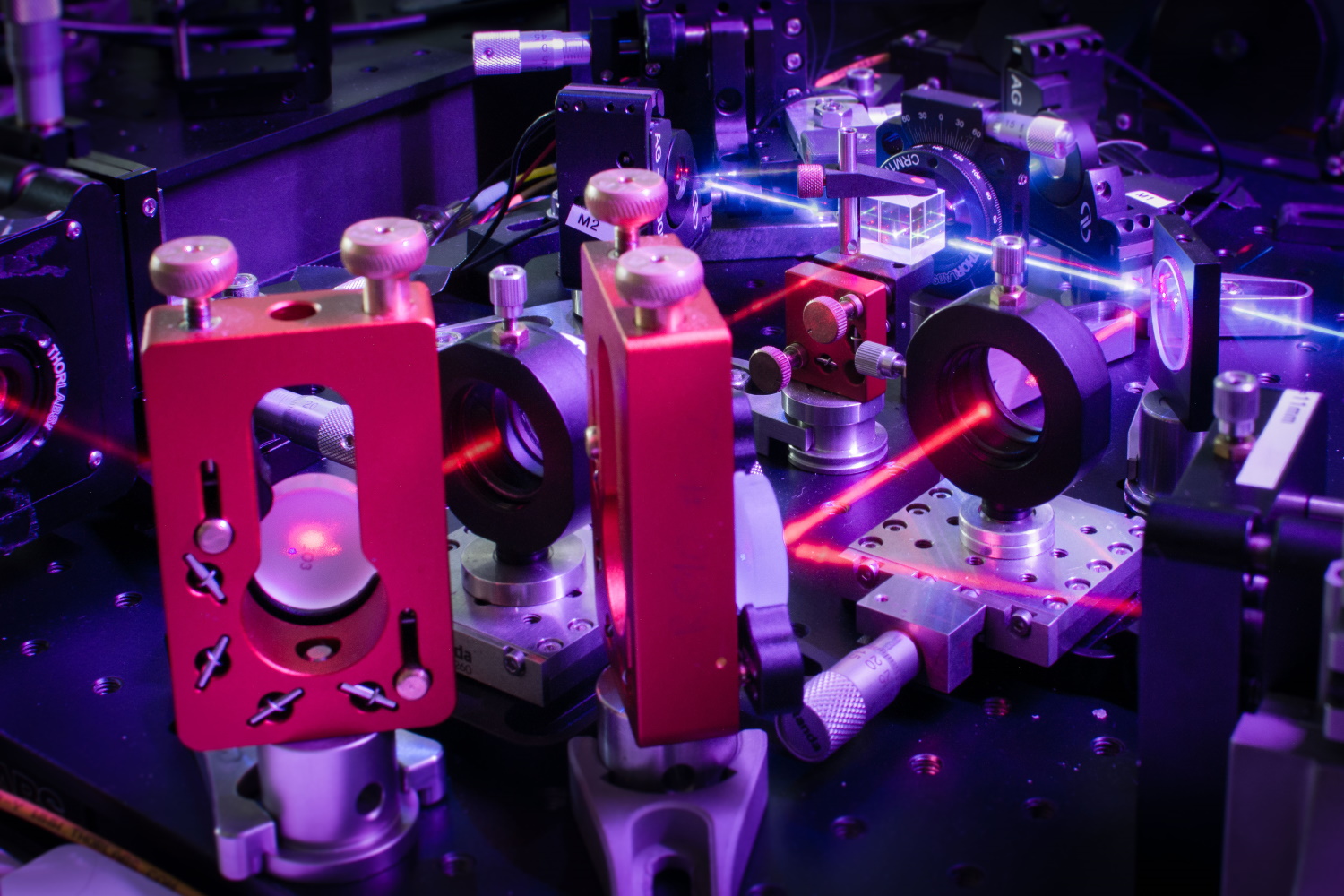
Laser Source by FSU Jena
The group has access experimental equipment including: ultra-bright entangled photon pair sources; Pulsed and cw- lasers at different wavelengths, Wavelength-division multiplexing technology, single photon detectors and time-tagging electronics, ultra-fast electro-optic modulators and pulse shaping technology, as well as spatial light modulators and adaptive optics. The figure depicts high-efficiency polarization-entangled photon source based on the process of spontaneous parametric down-conversion in a second-order nonlinear crystal. Entanglement in polarization is achieved by bi-directional pumping of a KTP crystal located at the center of a Sagnac Loop with a blue pump laser. Photon pairs produced at the center of the Sagnac loop are superimposed on a polarizing beam splitter, resulting in high-fidelity polarization entanglement (routinely >0.99 state fidelity). A red alignment laser is coupled into the collection fibers to indicate the output modes of the source. Ongoing research is focused on entanglement in photon number statistics, high-dimensional spatial-, and frequency modes, as well as simultaneous entanglement in multiple degrees of freedom (hyper-entanglement).
Laser Source by FSU Jena
The group has access experimental equipment including: ultra-bright entangled photon pair sources; Pulsed and cw- lasers at different wavelengths, Wavelength-division multiplexing technology, single photon detectors and time-tagging electronics, ultra-fast electro-optic modulators and pulse shaping technology, as well as spatial light modulators and adaptive optics. The figure depicts high-efficiency polarization-entangled photon source based on the process of spontaneous parametric down-conversion in a second-order nonlinear crystal. Entanglement in polarization is achieved by bi-directional pumping of a KTP crystal located at the center of a Sagnac Loop with a blue pump laser. Photon pairs produced at the center of the Sagnac loop are superimposed on a polarizing beam splitter, resulting in high-fidelity polarization entanglement (routinely >0.99 state fidelity). A red alignment laser is coupled into the collection fibers to indicate the output modes of the source. Ongoing research is focused on entanglement in photon number statistics, high-dimensional spatial-, and frequency modes, as well as simultaneous entanglement in multiple degrees of freedom (hyper-entanglement).
On-chip quantum homodyne detectionsystem
We will implement a single-photon counting quantum homodyne detection system with superior timing and low-noise performance on a silicon chip. A key feature of the SURQUID QHD system will be inherent stability for interferometric sensing applications and a high level of system integration. Using nanophotonic circuit design, we will achieve scalability of our detection platform that will be fabricated in a semiconductor industry fab. Operating in the telecom C-band, our QHD system that will provide enhanced sensitivity with SNSPD multi-detector architectures. The nanophotonic chip will integrate arrayed waveguide gratings, balanced directional couplers and waveguide coupled SNSPDs to realize the QHD scheme. To exploit quantum sensitivity in lidar, we will operate the QHD system on two wavelengths in parallel with ultrafast waveguide-integrated SNSPDs featuring <3 ps timing accuracy (jitter), which allows for a “coarse” determination of the position of an object to within 1mm via time-of-flight (TOF) measurements. Within the TOF measured spatial uncertainty, precision distance detection will be carried out using quantum homodyne detection. For 3D surface profiling we will implement a scanning beam detection system where each pixel under test is separately assessed with the QHDS. The photons returning from the target will be couple into nanophotonic waveguides via efficient 3D optical interfaces where they are mixed with a local oscillator field and recording with high-speed, low-noise SNSPDs. The electrical detector response will be processed with novel time to digital (TDC) application specific integrated circuits (ASIC) that allow to take advantage of the high timing accuracy and fast 2D scan rates realized with our SNSPDs.
On-chip quantum homodyne detectionsystem
Differing from today’s single photon sources, which have emerged primarily from quantum optics applications, our novel chip-based SURQUID ECS source will provide non-classical states which can be employed outside a laboratory setting. The integrated ECS source will provide key technological advances beyond the quantum sensing application, e.g. For continuous variable quantum computation, gravitational interferometry, and quantum enhanced precision measurements. We will realize an optical parametric amplifier (OPA) pumped from a frequency-doubled pulsed C-band laser. The corresponding wavelengths (~1550 nm) are an optimal compromise between ranging resolution, high atmospheric transmittance,
and interfacing with nanophotonic circuits and waveguide-coupled superconducting nanowire single-photon detectors (SNSPDs). Depending on the channel loss the source will be tuned via the pump parameter settings and fast switching to yield coherent states (CS), N00N-states, non-Gaussian entangled (or MM’) states or entangled coherent states (ECS). Within the project all these states will be experimentally verified (e.g., via violation of Bell inequalities or similar entanglement criteria). The source will be implemented in a portable form factor (prototype) for deployment in the field.
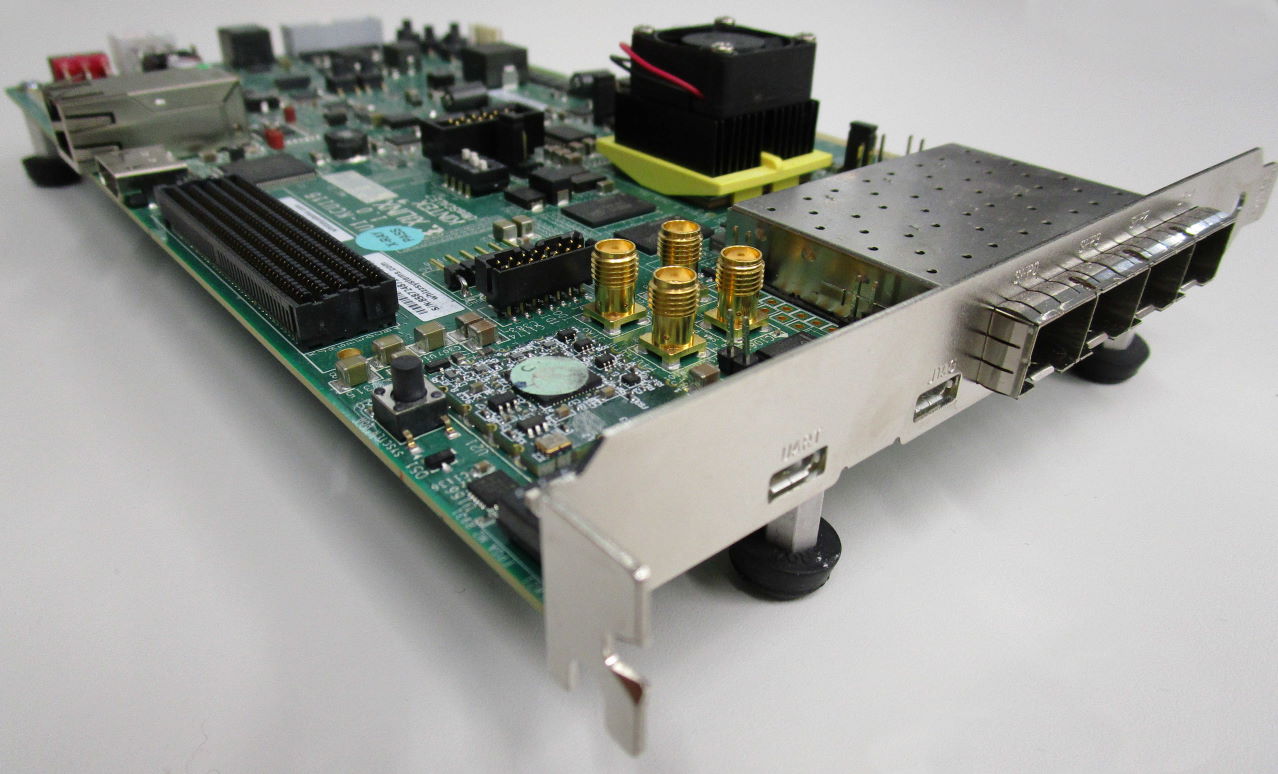
Read-Out Board for Processing SNSPD Signals with Ultimate Timing Resolution by Swabian Instruments
By reducing the FPGA’s structure size down to 16nm the company is able to further improve the resolution of their TDC (Time-to-Digital converter) technology. Existing evaluation tools allow them to research faster and focus on their development strengths. Click here for more information.
Read-Out Board for Processing SNSPD Signals with Ultimate Timing Resolution by Swabian Instruments
By reducing the FPGA’s structure size down to 16nm the company is able to further improve the resolution of their TDC (Time-to-Digital converter) technology. Existing evaluation tools allow them to research faster and focus on their development strengths. Click here for more information.
LiDAR
Multi-scale quantum imaging from 100 mm to 100 km distances: Practical application of the SURQUID platform for quantum enhanced lidar on a logarithmic length scale will provide first steps towards technologically relevant use. Multi-scale quantum imaging will exploit quantum enhancement for optical metrology. Objective 3 is application driven and will provide the ingredients for knowledge transfer and direct engagement with industry. Within the range determined from the TOF-measurement (O2) we will scan the source wavelength (phase) and interfere the photons returning from the remote object with a reference field. A photon counting measurement over a fixed time interval as function of the phase delay in the reference arm will then yield super-resolved interference fringes beating the Rayleigh limit by a factor of 2*pi*N^(-1), where N is the number of returning photons. Additional surface profiling will be realized by rasterizing the object using a MEMS device and exploiting angular super-resolution. Exploiting quantum technologies for lidar will not only push sensitivity beyond the classical limit but will open up new avenues for embedding complementary QHD concepts in current measurement systems. Beyond the fundamental proof of concept of quantum enhanced lidar, the SURQUID system will provide a practical implementation with a demonstrator system. The consortium counts with an industrial partner (IBEO), who is a leader in Lidar for the automotive sector and will enable transfer of the technology into real world applications that benefit from multi-scale quantum sensing with quantum enhanced sensitivity and scanning capabilities.
LiDAR
Multi-scale quantum imaging from 100 mm to 100 km distances: Practical application of the SURQUID platform for quantum enhanced lidar on a logarithmic length scale will provide first steps towards technologically relevant use. Multi-scale quantum imaging will exploit quantum enhancement for optical metrology. Objective 3 is application driven and will provide the ingredients for knowledge transfer and direct engagement with industry. Within the range determined from the TOF-measurement (O2) we will scan the source wavelength (phase) and interfere the photons returning from the remote object with a reference field. A photon counting measurement over a fixed time interval as function of the phase delay in the reference arm will then yield super-resolved interference fringes beating the Rayleigh limit by a factor of 2*pi*N^(-1),
where N is the number of returning photons. Additional surface profiling will be realized by rasterizing the object using a MEMS device and exploiting angular super-resolution. Exploiting quantum technologies for lidar will not only push sensitivity beyond the classical limit but will open up new avenues for embedding complementary QHD concepts in current measurement systems. Beyond the fundamental proof of concept of quantum enhanced lidar, the SURQUID system will provide a practical implementation with a demonstrator system.